Expanding ‘little brain’ may have powered dinosaur flight
The cerebellum swelled in size before flight evolved among modern birds’ dinosaur ancestors, according to a new comparison of fossilized skulls and living birds.
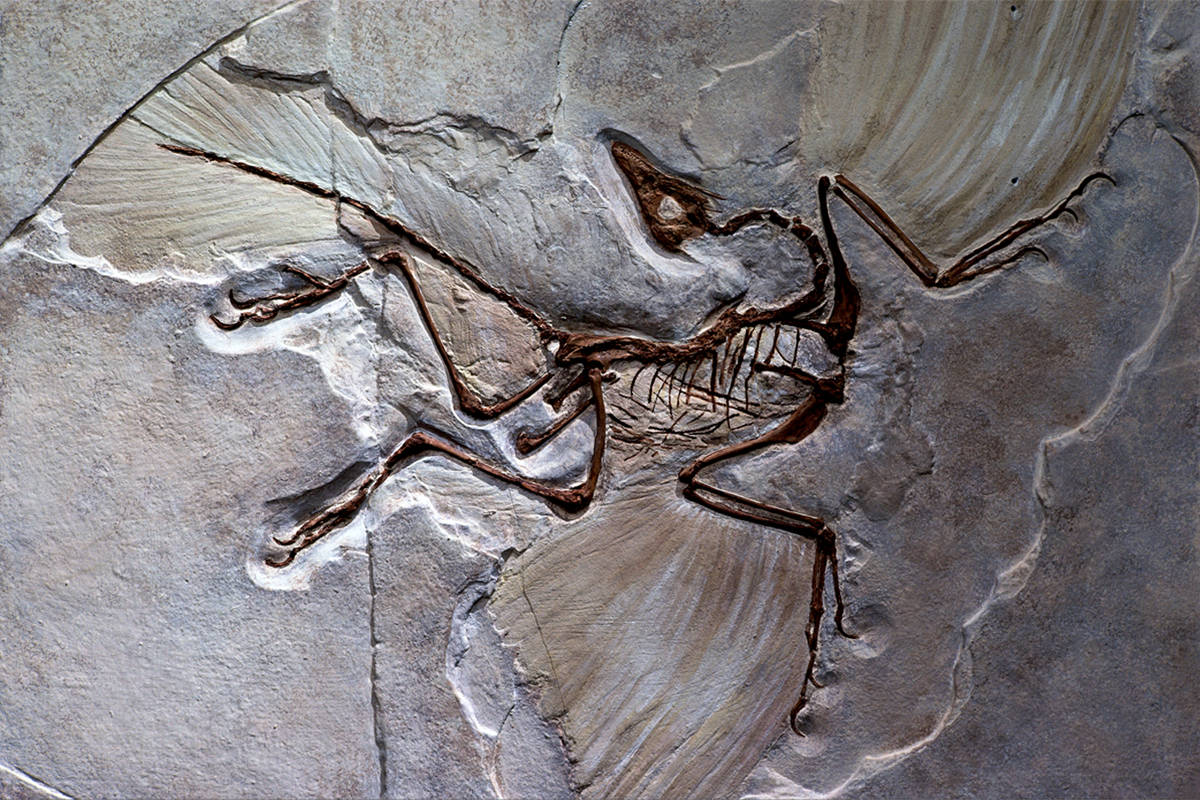
When the first known flying dinosaurs took to the skies some 150 million years ago, the evolutionary leap relied on adaptations to their nervous system. The changes remained a mystery, though, because of the paucity of fossilized neural tissue. Now fresh clues have emerged from a study that started with the long-gone dinosaurs’ living kin: the common pigeon, Columba livia.
Flight taps neural pathways involving the pigeon’s cerebellum, the new works shows, which prompted study investigator Amy Balanoff and her team to look specifically at that structure in digital brain “endocasts,” created by CT scanning fossilized dinosaur skulls.
“The birds can help us look for certain things within these extinct animals,” says Balanoff, assistant professor of evolutionary biology at Johns Hopkins University. “Then these extinct animals can tell us about the evolutionary history leading up to living birds.”
An analysis of the endocasts — from 10 dinosaur specimens dating to between 90 and 150 million years ago — revealed that the volume of the cerebellum expanded in birds’ closest relatives, but not in more distant ones. And at some point, the cerebellum began folding — instead of growing — to accommodate more neurons within a fixed cranial space, Balanoff says.
The results suggest that the cerebellum was “flight-ready before flying,” says Crístian Gutiérrez-Ibáñez, an evolutionary biology research associate at the University of Alberta who was not involved in the study. “So the question is, why did dinosaurs get such a big cerebellum?”
T
o visualize how a pigeon’s brain changes between rest and flight, Balanoff and her collaborators first teamed up with Paul Vaska, professor of radiology at Stony Brook University, to use positron emission tomography (PET) for whole-brain imaging.PET captures images of an injected radioactive tracer as it emits gamma rays. Vaska’s group used a common tracer called fluorodeoxyglucose (FDG), a radioactive glucose analog, as a proxy for neural activation: Neurons that are highly metabolically active take up the most FDG and thus return the brightest signal.
The pigeons received an FDG injection and then sat still on a perch or flew between perches for several minutes at a time. About an hour later, they were anesthetized and scanned. Because FDG is non-metabolizable and irreversibly taken up by cells, Vaska says, the scans provided a snapshot of what was happening when the birds were sitting or flying, despite the delay.
“The cerebellum just lights up when they fly,” Balanoff says, which wasn’t surprising because it integrates sensory information to plan and coordinate movement. “But just being able to look at it and actually say, ‘Yes, this is the region that’s most active during flight,’ is something that hasn’t been done before.”
Within the cerebellum, the strongest neural activation lit up at the ends of the birds’ two optic flow pathways, which relay information about visual changes across its retina as it moves through its environment. The most active pathway terminated in the oculomotor cerebellum, and the other terminated in the vestibular cerebellum. The results were published in January in Proceedings of the Royal Society B.
“I would have thought there would be more activity in the vestibular cerebellum,” the pathway involved in translational and rotational visual motion, than in the oculomotor pathway, which is thought to be important for steering and moving through a cluttered environment, given that the birds were simply flying between perches with no obstacles, says Andrea Gaede, assistant professor of sensory neurobiology at the University of London Royal Veterinary College, who was not involved with the study. Complicating the flight path or adding more elaborate visual stimuli could help tease out the contributions of each of the optic flow pathways, she says. Studying other bird species could also reveal which neural pathways are broadly conserved signatures of flight.
For all of the cerebellum’s involvement in modern flight, other types of locomotor changes, such as bipedalism, could have independently required its growth in size and complexity as birds evolved, Gutiérrez-Ibáñez says.
To better understand which pathways are truly flight-specific, Balanoff says her group plans to compare cerebellar activity between walking and flying birds.
More fossil specimens will help “better pinpoint where exactly these transformations are taking place,” she says. “It’s kind of amazing that you could look at what the brain would have looked like in something that lived 90 million years ago.”
Explore more from The Transmitter
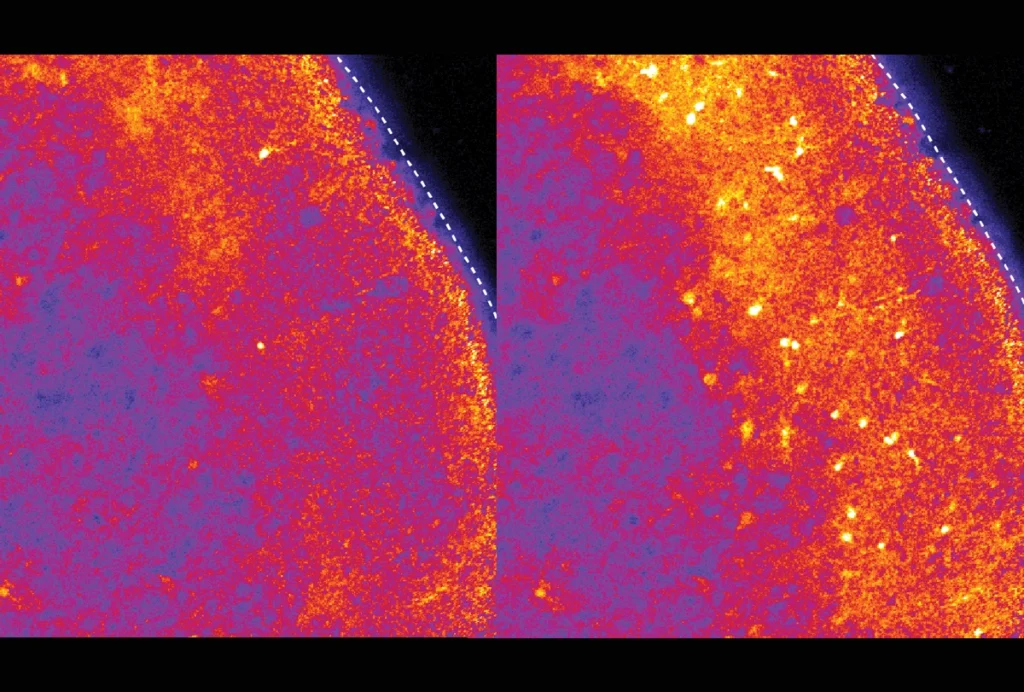
RNA drug corrects calcium signaling in chimeric model of Timothy syndrome
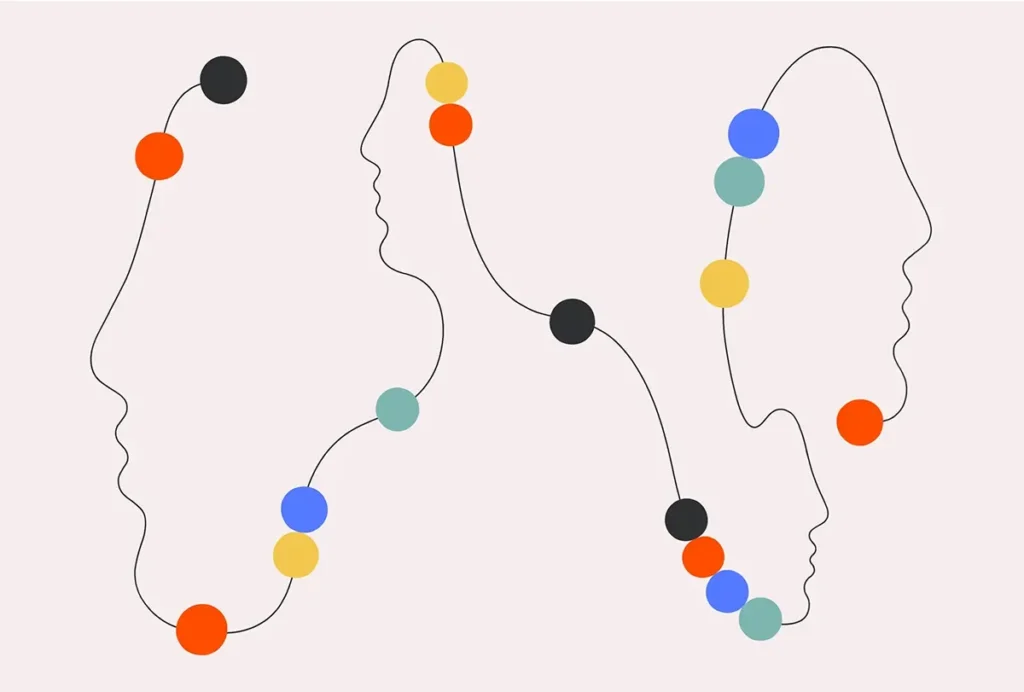